Nanomaterials - Buckyball
20.10The structure of C60 is a truncated (T = 3) icosahedron, which resembles a football of the kind made of twenty hexagons and twelve Pentagon, with a carbon atom at the nodes in each polygon and a bond along each edge of polygon.
Van der Waals diameter of a C60 molecule is about 1 nanometer (nm). The core of the core diameter of a C60 molecule is about 0.7 nm.
The C60 molecule has two bond lengths. The 6:6 ring bonds (between two hexagons) can be considered "double bonds" and are shorter than the 6:5 bonds (between a hexagon and a pentagon). Its average bond length is 1.4 angstroms.
Boron buckyball
A new type of bucky ball uses boron atoms instead of the usual carbon has been predicted and described by researchers at Rice University. B-80 structure is expected to be more stable than the C 60 bucky ball. One reason for this, which the researchers is that the B-80 is actually more like the original geodesic dome structure popularized by Buckminster Fuller, using triangles rather than hexagons. But this work has been the subject of much criticism by quantum chemists As it was concluded that the expected Ih vibrationally symmetric structure was unstable and the resulting cage undergoes a spontaneous symmetry breaking gives a puck cage with rare Th symmetry (symmetry in volleyball) The number of six rings of carbon in this molecule is 20 and the number of five member rings is 12.Variations of buckyballs
Another fairly common buckminsterfullerene is C70,but fullerenes with 72, 76, 84 and even up to 100 carbon atoms are commonly obtained.
In mathematical terms, the structure of a fullerene is a trivalent convex polyhedron with pentagonal and hexagonal faces. In graph theory, the term fullerene refers to any 3-regular, planar graph with all faces of size 5 or 6 (including the external face). It follows from Euler's polyhedron formula, |V|-|E|+|F| = 2, (where |V|, |E|, |F| indicate the number of vertices, edges, and faces), that there are exactly 12 pentagons in a fullerene and |V|/2-10 hexagons.
Nanomaterials - Variations
20.06Since the discovery of fullerenes in 1985, structural variations on fullerenes have evolved well beyond the individual clusters themselves. Examples include:
- buckyball clusters: smallest member is C 20 (unsaturated version of dodecahedrane) and the most common is C 60;
- nanotubes: hollow tubes of very small dimensions, having single or multiple walls; potential applications in electronics industry;
- megatubes: larger in diameter than nanotubes and prepared with walls of different thickness; potentially used for the transport of a variety of molecules of different sizes;
- polymers: chain, two-dimensional and three-dimensional polymers are formed under high pressure high temperature conditions
- nano"onions": spherical particles based on multiple carbon layers surrounding a buckyball core; proposed for lubricants;
- linked "ball-and-chain" dimers: two buckyballs linked by a carbon chain;
- fullerene rings
Nanomaterials - Fullerene
19.57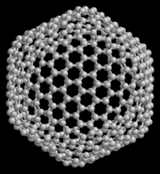
Full Clean is a family of carbon allotropes, molecules composed entirely of carbon in the form of a hollow sphere, ellipsoid, tube or plane. Spherical fuller's also known as bucky balls, and cylindrical them called carbon nanotubes or buckytubes. Grapher is an example of a planar format full clean sheets. Fuller's is similar in structure to graphite, which consists of stacked sheets of linked hexagonal rings, but may also contain pentagonal (or sometimes heptagonal) rings that would prevent a sheet from the planar format.
The full clean was discovered in 1985 by Robert Curl, Harold Kroto and Richard Smalley at the University of Sussex and Rice University who named it after Richard Buckminster Fuller, whose geodesic domes similar.
Prediction and discovery

Minute quantities of fuller's, in the form of C60, C70, C76 and C84 molecules that are produced in nature, hidden in soot and formed by lightning discharges in the atmosphere. Recently Buckminsterfullerenes was found in a family of minerals known as Shungites in Karelia, Russia. [citation needed]
The existence of C60 was predicted by Eiji Osawa of Toyohashi University of Technology in a Japanese magazine in 1970. He noticed that the structure of a molecule corannulene was a subset of a soccer-ball shape, and he put forward the hypothesis that a full ball shape could also exist. His idea was reported in Japanese magazines, but did not reach Europe or the USA.
Naming
Buckminsterfullerene (C60) was named after Richard Buckminster Fuller, a noted architectural modeler who popularized the geodesic dome. Since buckminsterfullerenes have a similar shape to that sort of dome, the name was thought to be appropriate. As the discovery of the fullerene family came after buckminsterfullerene, the shortened name 'fullerene' was used to refer to the family of fullerenes.
Chemical Processing of Ceramics
05.22_Fundamental Concept_
_Size Concerns_
_Materials Used_
_Chemical Processing of Ceramics_
In the treatment of fine ceramic, the irregular particle sizes and types in a typical powder often lead to uneven packing morphologies that result in packing density variations in the powder compact. Uncontrolled agglomeration of powders due to attractive van der Waals forces may also give rise to micro structural inhomogeneities.
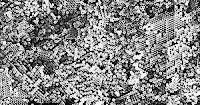
Furthermore, any fluctuation in packing density of the compact as it is prepared for the oven frequently is amplified during the sintering process which gives inhomogeneous densification. Some pores and other structural defects associated with density variations have been shown to play a negative role in the sintering process of growing and reducing the end-point densities.Differential stresses arising from inhomogeneous densification has also been shown to result in the propagation of internal cracks, and thus is the strength-controlling defects.
It would therefore be desirable to treat a material in a way that is physically consistent in terms of distribution of components and porosity instead of using the particle size distributions that will maximize the green density. Containment of a uniformly dispersed collection of strongly interacting particles in suspension requires complete control of particle-bound particle size interactions. Monodisperse colloids offer this potential.
Materials used in nanotechnology
05.21_Fundamental Concept_
_Size Concerns_
_Materials Used_
_Chemical Processing of Ceramics_
Materials referred to as "nanomaterials" generally fall into two categories: fullerenes, and inorganic nanoparticles.
Fullerenes
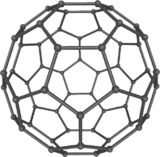
For the last decade, chemical and physical properties of fuller's been a hot topic in research and development, and will likely continue to be too long. In April 2003 the fuller was under study for potential medicinal use: binding specific antibiotics to the structure of resistant bacteria and even target certain cancer cells such as melanoma. From the October 2005 issue of chemistry and biology, contains an article describing the use of fuller's as light-activated antimicrobial agents. In nanotechnology, heat resistance and superconductivity are among the properties attract intense research.
A common method used to produce fuller agree to send a large current between two nearby graphite electrodes in an inert atmosphere. The resulting carbon plasma arc between the electrodes cools into sooty residue from which many fuller stocks can be isolated.
There are many calculations that have been performed using ab-initio quantum methods used for the fullernes. By DFT and TDDFT methods can achieve IR, raman and UV spectra. The results of these calculations can be compared with experimental results.
Nanoparticles
Nanoparticles or nanocrystals made of metals, semiconductors or oxides are of particular interest for their mechanical, electrical, magnetic, optical, chemical and other properties. Nanoparticles have been used as quantum dots and as chemical catalysts.Nanoparticles are of great scientific interest because they are actually a bridge between bulk materials and atomic or molecular structures. A bulk material should have constant physical properties regardless of its size, but at the nano-scale this is often not the case. Size-dependent properties are observed such as quantum confinement in semiconductor particles, surface plasmon resonance in some metal particles and superparamagnetism in magnetic materials.
Nanoparticles exhibit a number of special properties compared to bulk material. For example, the bending of bulk copper (wire, tape, etc.) arising from the movement of copper atoms / clusters at about 50 nm scale. Copper nanoparticles smaller than 50 nm are considered super hard materials that do not exhibit the same malleability and ductility as bulk copper. The change in properties is not always desirable. Ferroelectric materials smaller than 10 nm can switch their magnetisation direction using room temperature thermal energy, thus making them useless for memory storage. Suspensions of nanoparticles are possible because of the interaction of the particle size surface with the solvent is strong enough to overcome differences in density, usually resulting in a material either sinking or floating in a liquid. Nanoparticles often have unexpected visual properties, because they are small enough to limit their electrons and produce quantum effects. For example gold nanoparticles appear deep red to black in solution.
They are often very high surface area to volume ratio of nanoparticles provides a tremendous driving force for diffusion, especially at high temperatures. Sintering is possible at lower temperatures and over shorter periods than for larger particles. This theory does not affect the density of the final product, though flow difficulties and the tendency of nanoparticles to make the cake complicated. The surface effects of nanoparticles also reduces the incipient melting temperature.
Size concerns
05.18_Fundamental Concept_
_Size Concerns_
_Materials Used_
_Chemical Processing of Ceramics_
Another concern is that the volume of an object decreases as the third power of its linear dimensions, but the area only decreases as its second power. This somewhat subtle and unavoidable principle has enormous consequences. For example, the power of a drill (or any other machine) is proportional to the quantity, while the friction of the drill's bearings and gears is proportional to their area. For a normal-size drill, the power of the device is enough to handily overcome any friction. But scaling down its length by a factor of 1000, for example, decreases its power by 10,003 (a figure of one billion) and reduce the friction by only 10,002 (a figure of "only" one million). Proportionately it has 1000 times less power per friction than the original drilling. When the original skid-to-power ratio was, say, 1%, which means less drilling will have 10 times as much friction as a force. Drill is useless.
For this reason, while super-miniature electronic products, integrated circuits are fully functional, the same technology can not be used to make working mechanical devices than the rates in which friction forces are beginning to exceed the available power. Although you can see microphotographs of delicately etched silicon gears, these devices are little more than curiosities with limited real world, for example in moving mirrors and shutters. The surface tension increases at roughly the same way, so magnifying the tendency for very small objects to stick together. This could possibly make any kind of "micro-factory" impractical: even if the robotic arms and hands can be scaled down, something they pick up will tend to be impossible to put down. The above being said, molecular evolution has resulted in working ciliary flag ella, muscle fibers and rotary engines in aqueous environments, all on the nanoscale. These machines take advantage of the increased frictional forces exist at the micro or nano scale. Unlike a bat or a propeller, which depends on the normal friction forces (friction forces perpendicular to the surface) to achieve momentum ciliary movement develop from excessive drag or laminar forces (frictional forces parallel to the surface) present on the micro-and nano. To build a meaningful "machines" at the nano level, the forces must be considered. We are faced with the development and design of intrinsically safe machinery rather than the simple representations of macroscopic them.
All scaling issues must be assessed carefully when evaluating nanotechnology for practical applications.
Nanomaterials
20.28_Fundamental Concept_
_Size Concerns_
_Materials Used_
_Chemical Processing of Ceramics_
Nano materials are materials with morphological features smaller than a tenth of a micrometer in at least one dimension. Despite the fact that there is no consensus on the minimum or maximum size of nanomaterials, some authors limit their size to as low as 1 to ~ 30 nm, a logical definition would place the nano level between microscale (0.1 micrometre) and atomic / molecular scale (about 0.2 nanometers). See Figure "Classification of nanostructured materials."
Fundamental concepts
One aspect of nanotechnology is huge ratio of surface area to volume present in many nanoscale materials that enable new quantum mechanical, such as "quantum size effect" where the electronic properties of solids have been modified by large reductions in particle size. This effect does not come into play by going from macro to micro dimensions. But it becomes pronounced when nanometer size range is reached. A number of physical properties also change with the change of macroscopic systems. New mechanical properties of nanomaterials is a topic of nanomechanics research. Catalytic activities also reveal new behavior in interaction with biomaterials.Nanotechnology can be seen as extensions of traditional disciplines towards the explicit consideration of these properties. In addition, traditional disciplines can again be interpreted as specific applications of nanotechnology. This dynamic Reciprocation of ideas and concepts contributes to the modern understanding of the area. Broadly speaking, nanotechnology is the synthesis and application of ideas from science and engineering towards the understanding and production of new materials and equipment. These products generally make copious use of physical characteristics associated with small weights.
As mentioned above, the materials are reduced to the nanoscale can suddenly show very different properties compared to what they exhibit on a general, so unique applications. For instance, opaque substances become transparent (copper); inert materials attain catalytic properties (platinum); stable materials turn combustible (aluminum); solids turn into liquids at room temperature (gold); insulators become conductors (silicon). Materials such as gold, which is chemically inert at normal scales, can serve as a potent chemical catalyst at the nanoscale. Much of the fascination with nanotechnology stems from these unique quantum and surface phenomena that matter exhibits at the nanoscale.
Nano Size powder particles (a few nanometers in diameter, also called nanoparticles) are potentially important in ceramics, powder metallurgy, the achievement of a uniform nanoporosity and similar programs. The strong tendency of small particles to form clumps ( "cake") is a serious technological problem that impedes such applications. But a series of dispersants such as ammonium citrate (aqueous) and imidazoline or Oleyl alcohol (nonaqueous) are promising solutions as possible additives for deagglomeration.